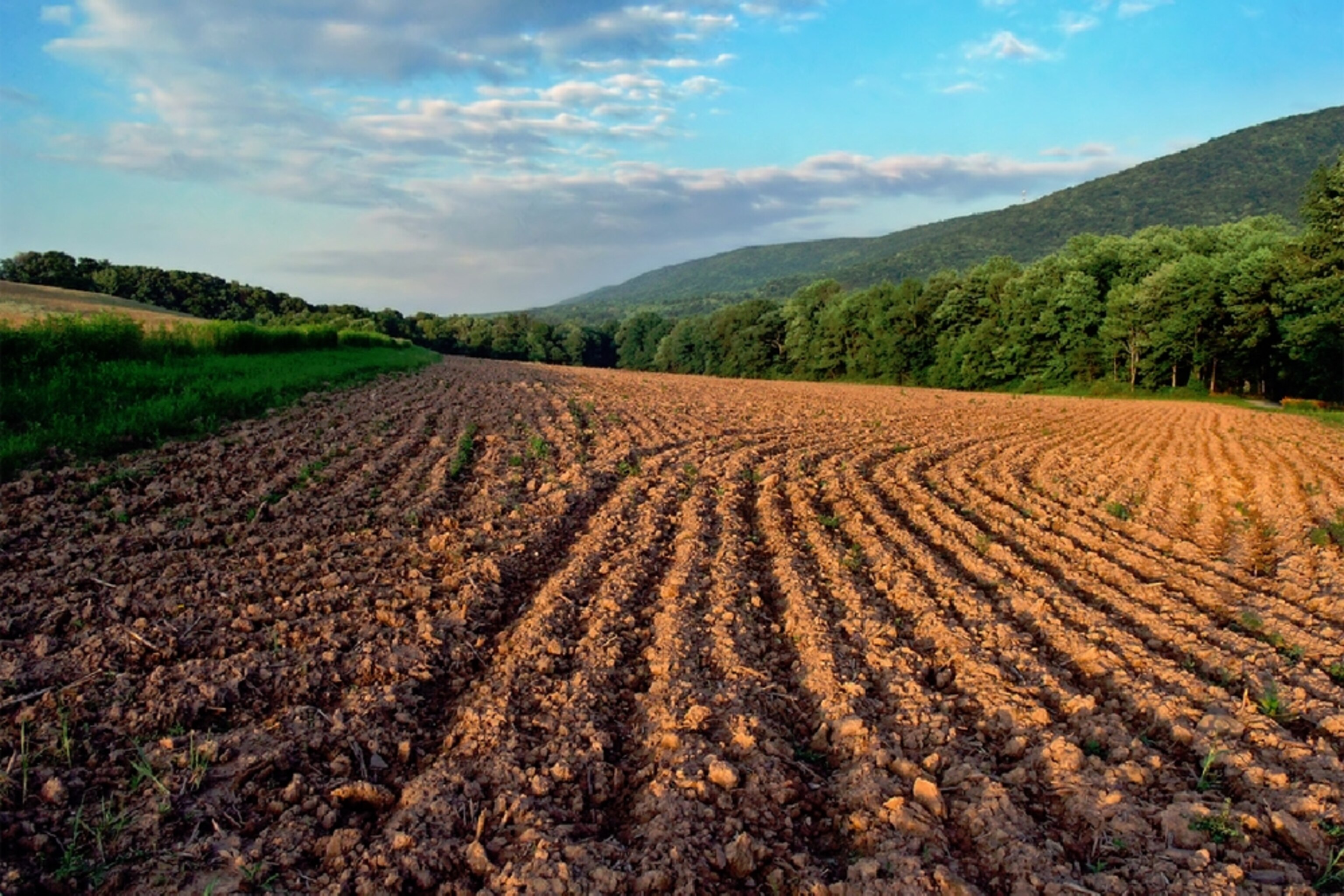
A New Antibiotic That Resists Resistance
The British chemist Lesley Orgel had a rule: Evolution is cleverer than you. Antibiotic-resistant bacteria have repeatedly proven him right.
Since humans started making antibiotics for ourselves in the 1940s, bacteria have evolved to counteract our efforts. They are now winning. There are strains of old foes that withstand everything we can throw at them. Meanwhile, our arsenal has dried up. Before 1962, scientists developed more than 20 new classes of antibiotics. Since then, they have made two.
More, hopefully, are coming. A team of scientists led by Kim Lewis from Northeastern University have identified a new antibiotic called teixobactin, which kills some kinds of bacteria by preventing them from building their outer coats. They used it to successfully treat antibiotic-resistant infections in mice. And more importantly, when they tried to deliberately evolve strains of bacteria that resist the drug, they failed. Teixobactin appears resistant to resistance.
Bacteria will eventually develop ways of beating teixobactin—remember Orgel—but the team are optimistic that it will take decades rather than years for this to happen. That buys us time.
Teixobactin isn’t even the most promising part of its own story. That honour falls on the iChip—the tool that the team used to discover the compound. Teixobactin is a fish; the iChip is the rod. Having the rod guarantees that we’ll get more fish—and we desperately need more.
Bacteria have been fighting each other for billions of years before we arrived, so environmental microbes are a rich source of potential new antibiotics. The problem is that 99 percent of them won’t grow in lab conditions. So, why not bring the environment into the lab?
That’s what the iChip does. It’s just a board with several holes in it. The team fill the holes by collecting soil, shaking it in water to release any microbes, heavily diluting the sample, mixing it with liquid agar, and pouring the agar into the iChip. The dilution ensures that each hole, now plugged by a disc of solid agar, contains just one bacterial cell. They then covered the discs in permeable membranes and dunked the whole board into a beaker of the original soil. The microbes are constrained to the agar, but they can still soak up nutrients, growth factors, and everything else they need from their natural environment. And thus, the ungrowable grows. “We have access to things that haven’t been seen before,” says Lewis.
“The method has the potential to be truly transformative, giving us access to a much greater diversity of environmental bacteria than previously imagined,” says Gautam Dantas from Washington University in St Louis.
Among these new microbes, the team found one species that kills staph bacteria efficiently. It belongs to an entirely new genus and is part of a group that’s not known for making antibiotics. They called it Eleftheria terrae. It yielded a compound—teixobactin—that could kill important rogues like the bacteria behind anthrax and tuberculosis, and Clostridium difficile (which causes severe diarrhoea). The team exposed some of these microbes to low levels of teixobactin for several weeks, to see if resistant strains would evolve. None did.
“I thought: Aw, damn it,” says Lewis. “We discovered a detergent.”
Counter-intuitively, if you see a total lack of resistance, it usually means that you’ve discovered a compound so toxic that it’s never going to work in an actual human. Hence: Lewis’s dismay. But when his team applied the drug to mammalian cells, it wasn’t toxic at all. It seemed safe, stable in blood, and capable of protecting mice from lethal doses of MRSA (drug-resistant staph). Things were looking up.
Losee Ling from NovoBiotic Pharmaceuticals and Tanja Schneider at the University of Bonn showed that teixobactin works by withholding two molecules—Lipid II, which bacteria need to make the thick walls around their cells, and Lipid III, which stops their existing walls from breaking down. When teixobactin is around, bacterial walls come crumbling down, and don’t get rebuilt.
The drug also sticks to parts of both Lipid II and Lipid III that are constant across different species of bacteria. It’s likely that these parts can’t be altered without disastrous consequences, making it harder for bacteria to avoid teixobactin’s double-punch. This might explain why it’s so hard to evolve resistance to the drug.
This won’t work on every bacterium. Many of them, like E.coli, Salmonella, and Helicobacter, have another membrane around their cell walls that can deflect teixobactin. So does E.terrae—the microbe that makes the drug in the first place. That’s actually a good thing. Lewis says that many of the resistance mutations that defuse antibiotics originate the microbes that produce those drugs—after all, they must protect themselves. But since E.terrae is impervious to teixobactin, it doesn’t need any such mutations. It has no countermeasure for other bacteria to borrow. “It started looking to us like a fool-proof case of no resistance,” he says.
The existing antibiotic vancomycin also works by sticking to Lipid II, albeit to a different part of the molecule that changes more from one microbe to the next. It took 30 years for bacteria to start resisting vancomycin, and Lewis hopes that teixobactin resistance will take even longer to appear.
“We are constantly in need of new antibiotics with novel mechanisms of action, especially ones that can evade known resistance mechanisms,” says Karen Bush from Indiana University. Teixobactin certainly fits that bill, but Bush is sceptical that it is as resistance-proof as it first appears. “Other agents have been studied in similar kinds of resistance selection studies as described in the paper. Although those drugs had no demonstrable resistance under that set of conditions, more stringent selection procedures resulted in detection of resistant strains,” she says.
Laura Piddock, a microbiologist at the University of Birmingham and leader of Antibiotic Action, adds that other environmental bacteria might harbour countermeasures to teixobactin. “To be sure that resistance to this new antibiotic is unlikely to occur in the clinical setting, bacteria isolated from the same environmental niche should be screened for teixobactin-resistance conferring genes,” she says in an email.
Meanwhile, Lewis’ team are doing more tests with teixobactin in other animals, with a view to eventually getting FDA approval. They’re also trying to tweak the compound and make it more soluble, which would allow them to give people higher doses. And, of course, he will continue to use the iChip to identify even more potential drugs.
Will we ever return to the glory days of antibiotic discovery?
“There’s no doubt in my mind that we’ll do exactly that,” he says.
Reference: Ling, Schneider, Peoples, Spoering, Engels, Conlon, Mueller, Schaberle, Hughes, Epstein, Jones, Lazarides, Steadman, Cohen, Felix, Fetterman, Millett, Nitti, Zullo, Chen & Lewis. 2015. A new antibiotic kills pathogens without detectable resistance. Nature. http://dx.doi.org/10.1038/nature14098
Go Further
Animals
- How can we protect grizzlies from their biggest threat—trains?How can we protect grizzlies from their biggest threat—trains?
- This ‘saber-toothed’ salmon wasn’t quite what we thoughtThis ‘saber-toothed’ salmon wasn’t quite what we thought
- Why this rhino-zebra friendship makes perfect senseWhy this rhino-zebra friendship makes perfect sense
- When did bioluminescence evolve? It’s older than we thought.When did bioluminescence evolve? It’s older than we thought.
- Soy, skim … spider. Are any of these technically milk?Soy, skim … spider. Are any of these technically milk?
Environment
- Are the Great Lakes the key to solving America’s emissions conundrum?Are the Great Lakes the key to solving America’s emissions conundrum?
- The world’s historic sites face climate change. Can Petra lead the way?The world’s historic sites face climate change. Can Petra lead the way?
- This pristine piece of the Amazon shows nature’s resilienceThis pristine piece of the Amazon shows nature’s resilience
- Listen to 30 years of climate change transformed into haunting musicListen to 30 years of climate change transformed into haunting music
History & Culture
- Meet the original members of the tortured poets departmentMeet the original members of the tortured poets department
- Séances at the White House? Why these first ladies turned to the occultSéances at the White House? Why these first ladies turned to the occult
- Gambling is everywhere now. When is that a problem?Gambling is everywhere now. When is that a problem?
- Beauty is pain—at least it was in 17th-century SpainBeauty is pain—at least it was in 17th-century Spain
Science
- Here's how astronomers found one of the rarest phenomenons in spaceHere's how astronomers found one of the rarest phenomenons in space
- Not an extrovert or introvert? There’s a word for that.Not an extrovert or introvert? There’s a word for that.
- NASA has a plan to clean up space junk—but is going green enough?NASA has a plan to clean up space junk—but is going green enough?
- Soy, skim … spider. Are any of these technically milk?Soy, skim … spider. Are any of these technically milk?
Travel
- How to see Mexico's Baja California beyond the beachesHow to see Mexico's Baja California beyond the beaches
- Could Mexico's Chepe Express be the ultimate slow rail adventure?Could Mexico's Chepe Express be the ultimate slow rail adventure?