For many paralyzed people, their problem is a communication gap. They can generate the signals in their brain require to control their muscles–to walk, to wash dishes, to weed a garden. But damage to their nervous system prevents those signals from reaching their destination.
Last year, in a feature I wrote for National Geographic about the brainfeature I wrote for National Geographic about the brainfeature I wrote for National Geographic about the brain, I recounted the work of scientists and engineers who are trying to bridge that gap. Their dream is to create a technology that reads signals from people’s brains and uses them to control machines. The machines might be robot arms that people could use to feed themselves, or computers to compose emails, or perhaps even exoskeletons that could enable people to walk.
Scientists have been investigating these brain-machine interfaces for decades, and in recent years they’ve made some impressive advances–some of which I described in my story. But it would be wrong to giddily declare that scientists have reached their goal. You need only look at this picture below to get a sense of how far we are from science-fiction dreams.
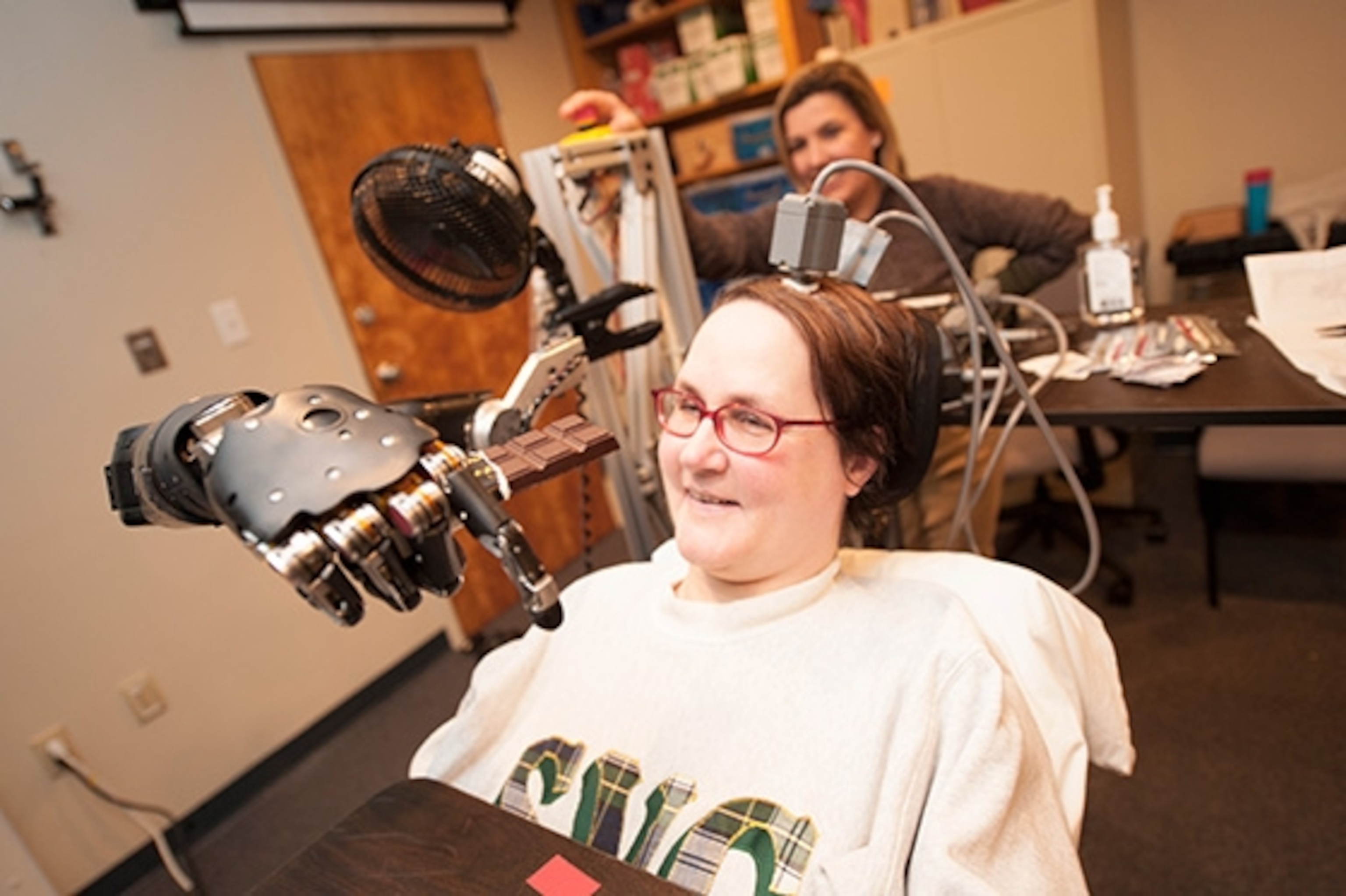
This woman, Jan Scheuermann, is at the forefront of brain-machine interface research. She volunteered to have an electrodes injected into the surface of her brain. Researchers at the University of Pittsburgh connected the electrodes to pedestals on top of her scalp. Cables can be attached to the pedestals; they connect to a computer and a power source.
Scheuermann and the scientists worked together to train the computer to recognize signals from her brain and use them to control a robot arm. In December 2012, Scheuermann made news by controlling the robot arm so well she could feed herself a bar of chocolate.
But this system was hardly ready for prime time. The electrode apparatus has to pass through a hole in a patient’s skull, creating the risk of infection. The cables tether the patient to bulky machines, which would make the whole system cumbersome rather than liberating.
In addition, the robot arm had plenty of room for improvement. It had seven degrees of freedom. Scheuermann could control its shoulder, elbow, and wrist joints. The hand, however, could only open and close. So Scheuermann had the same kind of dexterity as if she wore a mitten.
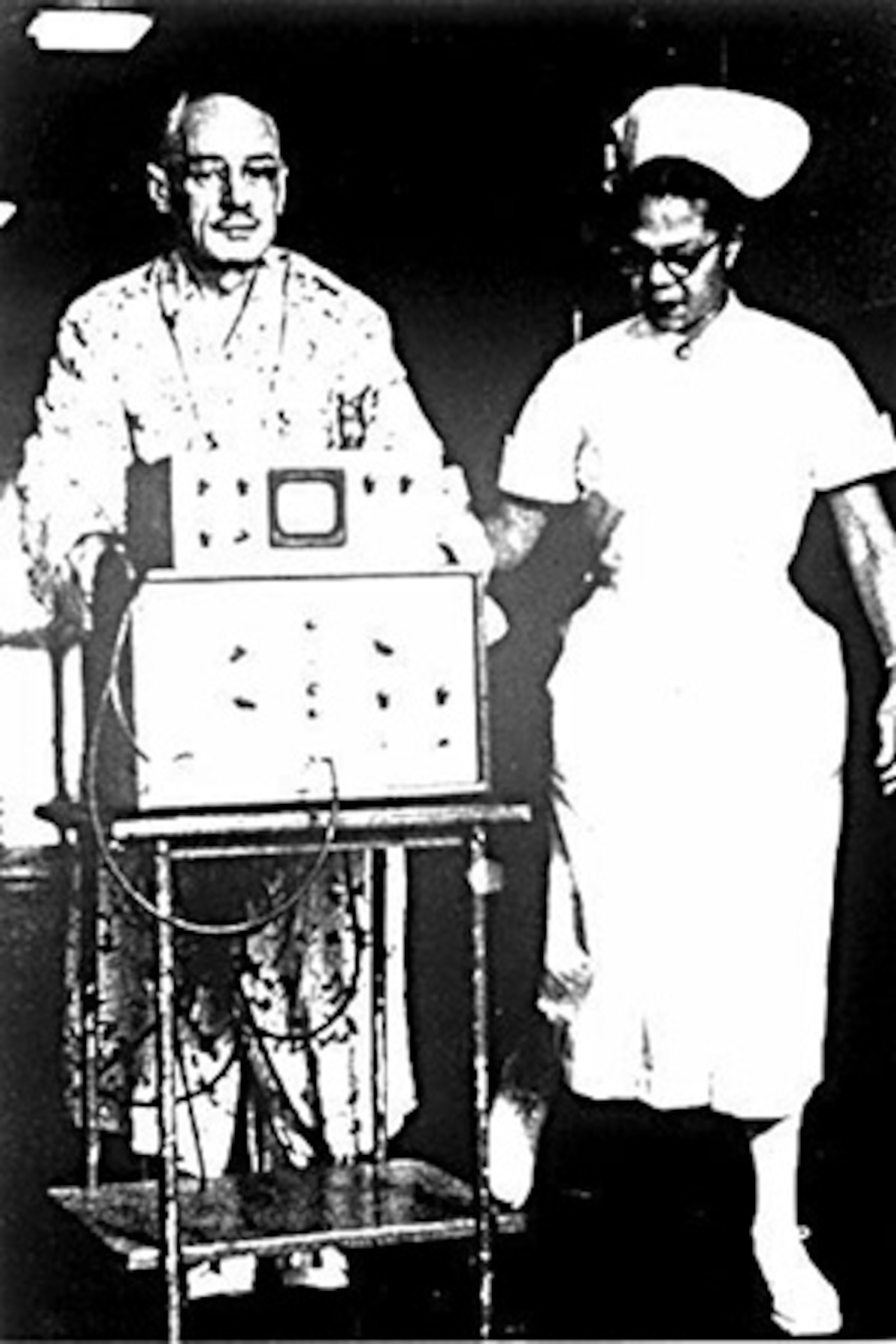
None of this was any reason to dismiss brain-machine interfaces as having reached a dead end. The history of pacemakers started out in much the same place. Today, people can walk around with pacemakers implanted in their chests without anyone around them having the slightest awareness that a device is regulating their heartbeat. Sixty years ago, however, the first pacemakers were enormous, cumbersome affairs. Implanted electrodes were tethered to wires that ran to big machines. Patients either had to lay next to the machines or trundle them around on a cart. The pacemakers were also relatively simple, delivering fixed patterns of electricity to the heart. Too often, they failed to keep the heart working.
In the 1960s, pacemakers became portable and battery-powered. They still needed external wires, but the wires now ran to a small box that a patient could carry on a belt. Finally, pacemakers disappeared into the body completely. In 2009, doctors began implanting pacemakers that not only had their own power supply but could also communicate medical information to doctors with a Wi-Fi connection to the Internet. Pacemakers also deliver more sophisticated signals to the heart, using algorithms to adjust their rhythms. If someone looked at the ungainly state of pacemakers in 1960 and declared them hopeless, they would have been profoundly wrong.
Two new studies are pushing brain-machine interfaces forward in the same way.
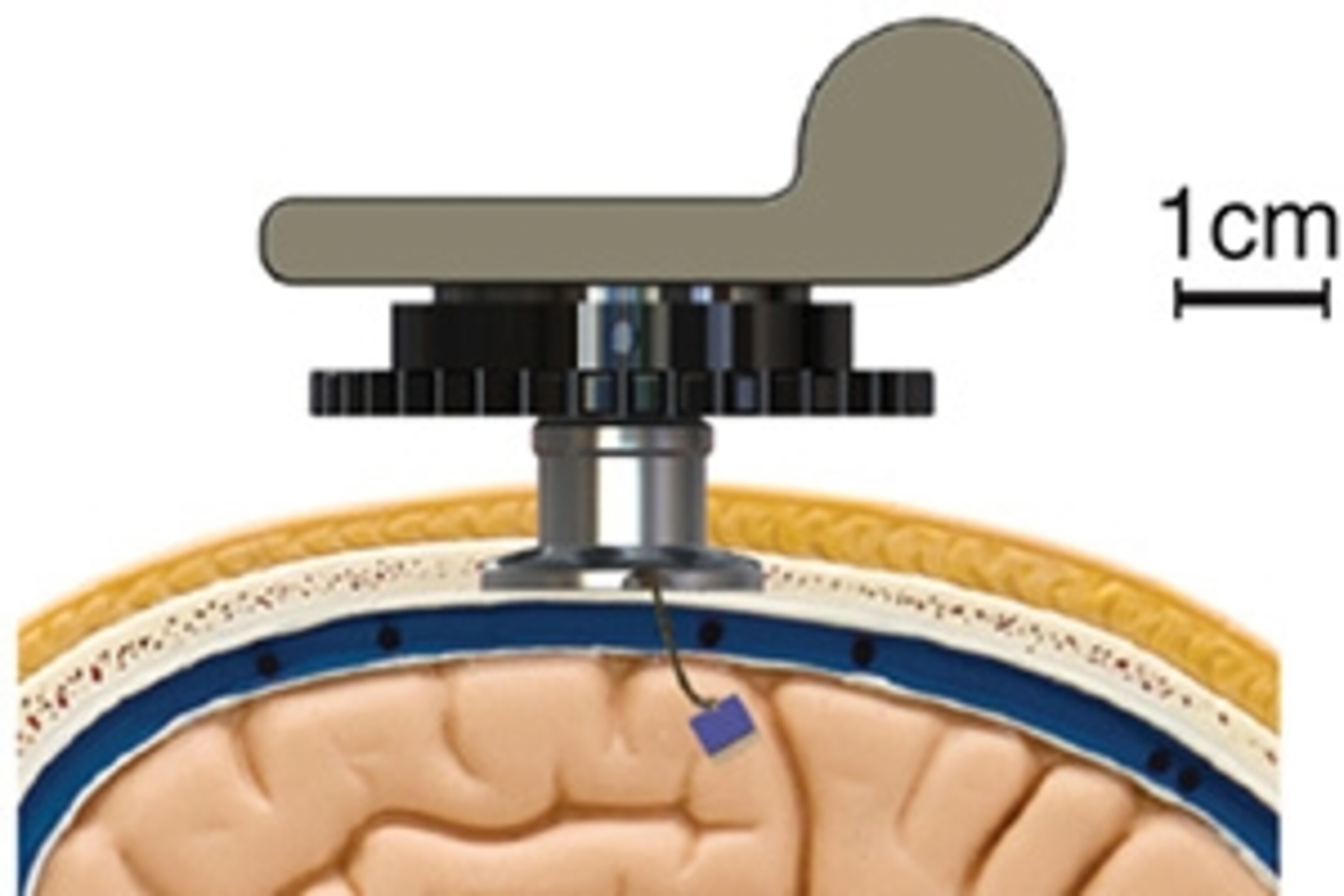
The first study advances the electrode end of the interface. A team of scientists led by Arto Nurmikko of Brown University developed an implant that requires no wires. The implant can pick up signals from 100 different electrodes. It contains microelectronics that can turn these signals into a Wi-Fi transmission broadcast at a rate of 200 Mb per second. The researchers implanted the device in monkeys and found that they could pick up signals from five yards away with a quality on par with signals delivered by cables. The monkeys went about their business freely, and the scientists could pick out signals they used to walk on a treadmill. When the monkeys fell asleep, the scientists could detect shifts in their brain waves. The whole apparatus runs for over two day straight on a double AA battery.
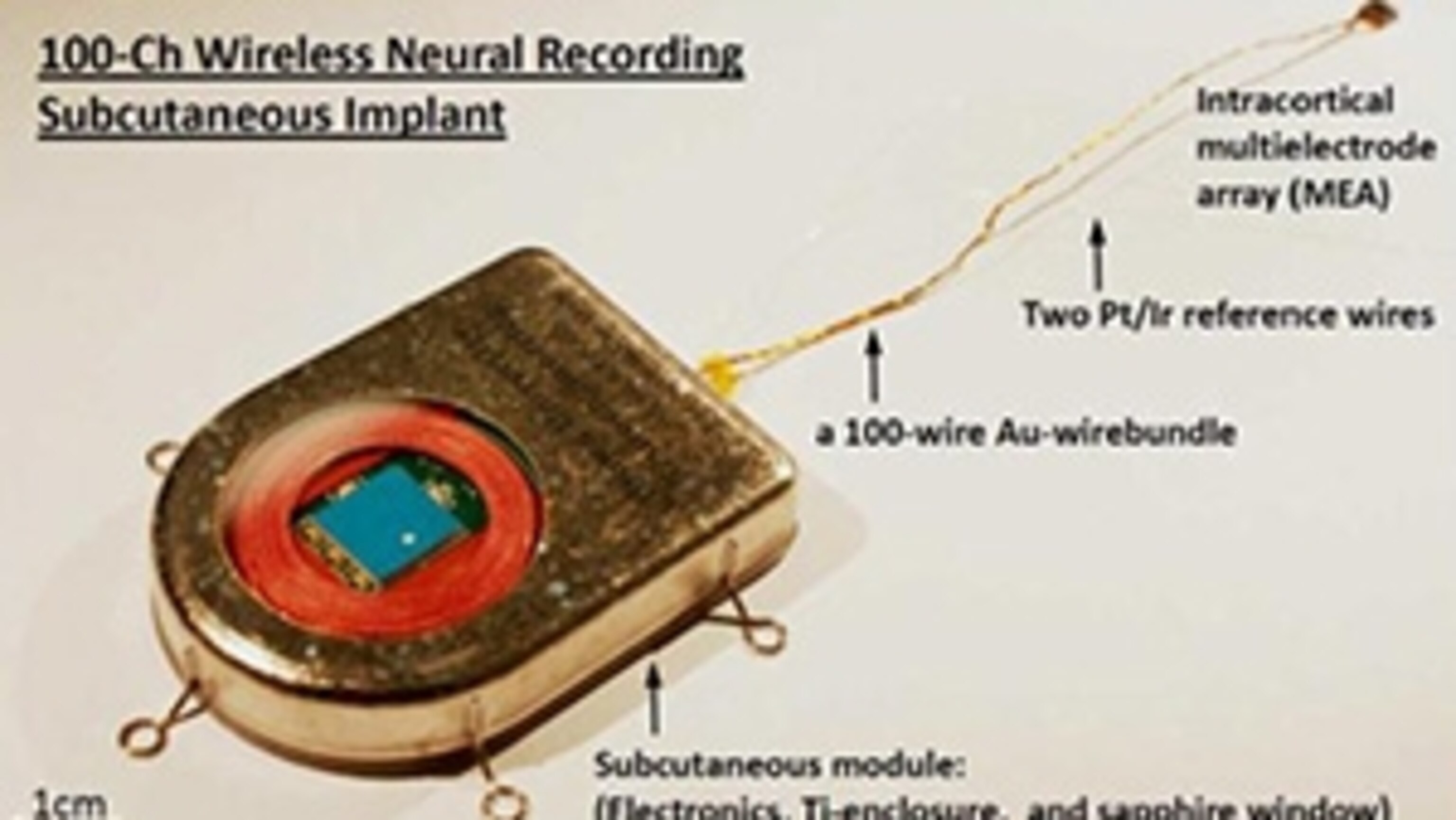
For now, this device will probably be most useful to researchers who study the behavior of animals. But Nurmikko and his colleagues are also learning lessons for the next generation of brain-machine interfaces for people. In another promising line of research, they have designed a prototype of a fully implantable device. The electrodes go in the brain, while the power source and transmitter sit atop the skull, below the scalp. In the future, scientists may be able to make new devices that take advantage of both studies–implants that can be sealed in the head, transmit a lot of data wirelessly, run efficiently on a long-lasting battery, and not heat up the way electronics sometimes do.
Meanwhile, at the other end of the interface, Scheuermann has been testing out a new and improved robot arm. The Pittsburgh team programmed four different positions that the hand could take, such as pinching the index and thumb together. The researchers had no idea if all those extra degrees of freedom would be too much for their interface to handle. Could it pick out signals in Scheuermann’s brain that were meaningful enough to make full use of the arm’s range of motion?
To train Scheuermann, the scientists had her start her practice on a virtual robot arm, which she used to grab virtual objects on a computer screen. The computer system learned how to recognize certain patterns of neuron signals as commands to change the shape of the robot hand. At the same time, Scheuermann’s own brain became more adept at controlling the robot arm, producing stronger signals. Finally, the scientists had Scheuermann try to pick up a number of different objects. Here’s a sampling of her successes:
Scheuermann, as the scientists had hoped, learned how to manage her new arm. It wasn’t a perfect education. Scheuermann sometimes failed to grab objects, and the scientists never managed to record a success on certain tasks, such as pouring water from one glass into another.
Still, the results were encouraging–and sometimes intriguing. The scientists found some groups of neurons that would fire together in distinctive patterns as Scheuermann moved the arm through all ten dimensions. In other words, these neurons weren’t limited to just bending the elbow or pinching a thumb. In the future, it may be possible to harness these flexible signals to make the arms even more proficient, and to fill the communication gap even more.
Go Further
Animals
- This fungus turns cicadas into zombies who procreate—then dieThis fungus turns cicadas into zombies who procreate—then die
- How can we protect grizzlies from their biggest threat—trains?How can we protect grizzlies from their biggest threat—trains?
- This ‘saber-toothed’ salmon wasn’t quite what we thoughtThis ‘saber-toothed’ salmon wasn’t quite what we thought
- Why this rhino-zebra friendship makes perfect senseWhy this rhino-zebra friendship makes perfect sense
Environment
- Your favorite foods may not taste the same in the future. Here's why.Your favorite foods may not taste the same in the future. Here's why.
- Are the Great Lakes the key to solving America’s emissions conundrum?Are the Great Lakes the key to solving America’s emissions conundrum?
- The world’s historic sites face climate change. Can Petra lead the way?The world’s historic sites face climate change. Can Petra lead the way?
- This pristine piece of the Amazon shows nature’s resilienceThis pristine piece of the Amazon shows nature’s resilience
- 30 years of climate change transformed into haunting music30 years of climate change transformed into haunting music
History & Culture
- When treasure hunters find artifacts, who gets to keep them?When treasure hunters find artifacts, who gets to keep them?
- Meet the original members of the tortured poets departmentMeet the original members of the tortured poets department
- When America's first ladies brought séances to the White HouseWhen America's first ladies brought séances to the White House
- Gambling is everywhere now. When is that a problem?Gambling is everywhere now. When is that a problem?
Science
- Should you be concerned about bird flu in your milk?Should you be concerned about bird flu in your milk?
- Here's how astronomers found one of the rarest phenomenons in spaceHere's how astronomers found one of the rarest phenomenons in space
- Not an extrovert or introvert? There’s a word for that.Not an extrovert or introvert? There’s a word for that.
Travel
- This striking city is home to some of Spain's most stylish hotelsThis striking city is home to some of Spain's most stylish hotels
- Photo story: a water-borne adventure into fragile AntarcticaPhoto story: a water-borne adventure into fragile Antarctica
- Germany's iconic castle has been renovated. Here's how to see itGermany's iconic castle has been renovated. Here's how to see it