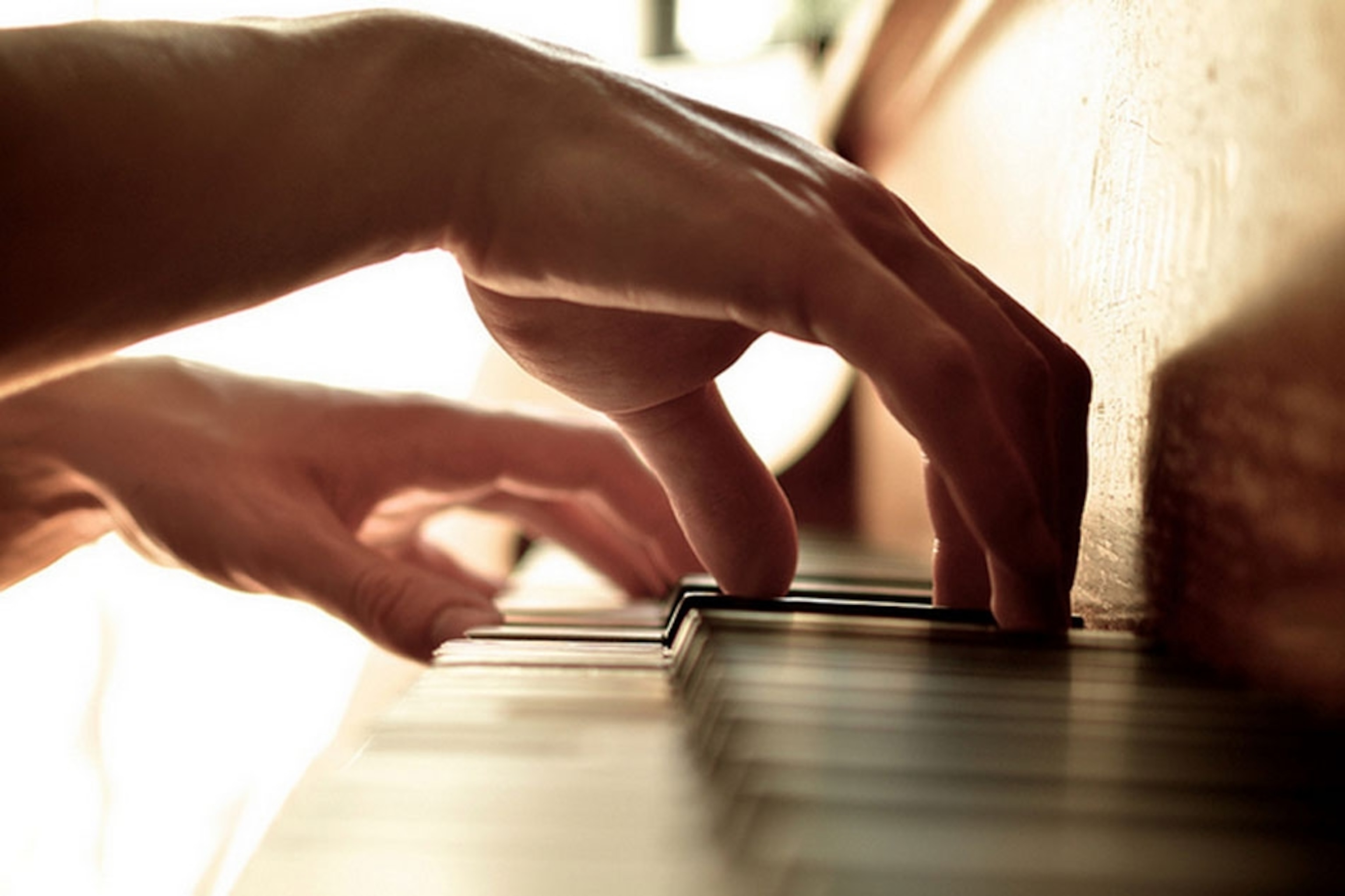
How Did You Get Five Fingers?
Your arms and toes began as tiny buds that sprouted from your sides when you were just a four-week-old embryo. By six weeks, these limb buds had grown longer and five rods of cartilage had appeared in their flattened tips. By week seven, the cells between the rods had died away, sculpting five small fingers or toes from once-solid masses of flesh.
Now, a team of scientists led by James Sharpe from the Centre for Genomic Regulation in Barcelona has discovered that these events are orchestrated by three molecules. They mark out zones in the embryonic hand where fingers will grow, and the spaces in between that are destined to die. Without this trinity, pianos and keyboards wouldn’t exist, jazz hands would be jazz palms, and giving someone the finger would be impossible.
These three molecules work in a way first envisioned by legendary English mathematician and code-breaker Alan Turing. Back in 1952, Turing proposed a simple mathematical model in which two molecules could create patterns by spreading through tissues and interacting with each other. For example, the first molecule might activate the second, while the second blocks the first. Neither receives any guidance about where to go; through their dance, they spontaneously organise themselves into spots or stripes.
Since then, many scientists have found that these Turing mechanisms actually exist. They’re responsible for a cheetah’s spots and an zebrafish’s stripes. For 30 years, people have also suggested that they could sculpt our hands and feet, but no one had found the exact molecules involved.
Sharpe knew that these molecules would need to show a striped pattern—they’d either be active in the bits that become the fingers or the areas in between. Sox9 seemed like the most promising candidate. It is activated in a striped pattern from a very early stage of development. It controls the activity of other genes and if you get rid of it, its underlings lose their neat periodic patterns.
By comparing cells where Sox9 is active or inactive, Jelena Raspopovic and Luciano Marcon found two other groups of genes—Bmp and Wnt—also formed striped patterns. Bmp rises and falls in step with Sox9 and both are active in the digits. Wnt is out of phase; it’s active in the gaps. The three molecules also affect each other: Bmp activates Sox9 while Wnt blocks it; and Sox9 blocks both of its partners.
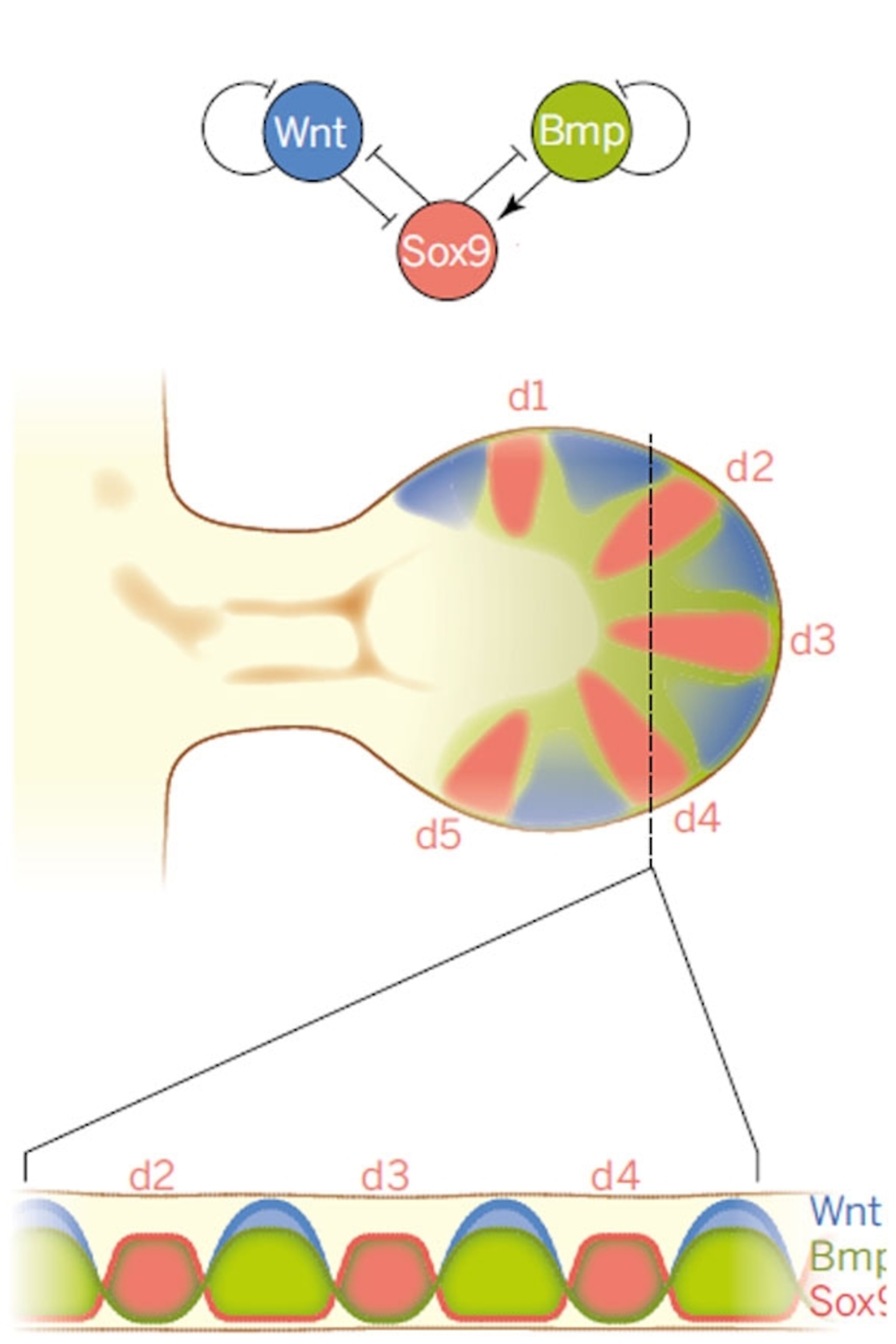
It looked like these were the molecules the team was searching for—not a pair, as Turing suggested, but a trinity. To confirm this, they created a simulation of a growing limb bud and showed that Sox9, Bmp and Wnt can organise themselves into a pattern of five stripes, by activating and blocking each other.
The team also used their simulation to predict what would happen if they removed each of the partners from the dance. If they took out Bmp, Sox9 activity also died away and the fingers didn’t form at all; instead, the virtual limb bud continued growing as a shapeless lump. If they took out Wnt, Sox9 became active everywhere and the spaces between the fingers disappeared. If they blocked Bmp and Wnt together, these effects partly cancelled each other out but the number of fingers decreased.
The team then checked these predictions by applying drugs that block Wnt and Bmp to isolated limb buds growing in Petri dishes. In every case, the reality matched the predictions.
There’s still a lot to discover, though. For example, I’ve used Bmp and Wnt as shorthands here—in reality, each represents a class of several molecules, and the team still needs to work out which specific member is part of the Turing trinity.
They also want to identify molecules that affect the trinity. One of these might be FGF, a protein that’s more concentrated at the fingertips than at the base of the hand. Sharpe thinks that it changes the relationships between the Turing trinity to widen the Wnt canyons between the Sox9/Bmp peaks. It effectively increases the wavelength of the fingers as you move to the tip of the hand. It might explain why your fingers are slightly splayed, rather than strictly parallel.
There’s also the most obvious question: why do we have five fingers and toes?
On one level, the answer depends on simple physical traits like how quickly the Turing molecules spread through the hand, how strongly the interact with each other, and how fast the limb bud grows. If the molecules diffuse more quickly, the gap between the fingers would be larger and you’d get fewer digits. If the limb bud grows 20 percent bigger and everything else stays the same, you suddenly have room for an extra digit—this is why around 1 in 500 people are born with an extra finger or toe.
These cases, known as polydactyly, show that there’s a lot of flexibility built into the Turing system. Change the parameters slightly, and you can change the numbers of fingers and toes. So why has evolution set these parameters so they almost always make five? It’s clearly possible to make more. Some people are born with more. Ernest Hemingway used to own a six-toed cat, whose descendants still live in the writer’s Florida home. And the first tetrapods (four-legged animals) to invade the land had anywhere up to eight toes per foot.
But the common ancestor of all mammals, birds, reptiles and amphibians had five, and we have stuck with that number. Many groups have lost digits, but five is still the basic number. A horse has a single toe on each foot but if you look at an early horse embryo, its limb buds have five little stripes of Sox9, just like ours.
Some might say that we never need more than five fingers, but that’s not true either. Pandas have adapted a wrist bone into a pseudo-thumb to help them grasp bamboo; they effectively have six fingers. Others think that it’s too hard to change the number of digits because the pertinent genes (like Sox9) control the development of other body parts. Mutations that give you more fingers might also screw up your heart or spine. But Sharpe doesn’t like this answer either. “It implies that the animal body plan is fairly locked, and obviously evolution happens,” he says.
So, why five? No one really knows. “It’s the ultimate meta-problem on top of everything,” says Sharpe. “I often say that if we understood why five, we’d probably understand everything.”
References: Raspopovic, Marcon, Russo & Sharpe. 2014. Bmp-Sox9-Wnt Turing network modulated by morphogen gradients. Science http://dx.doi.org/10.1126/science.1252960
Go Further
Animals
- How can we protect grizzlies from their biggest threat—trains?How can we protect grizzlies from their biggest threat—trains?
- This ‘saber-toothed’ salmon wasn’t quite what we thoughtThis ‘saber-toothed’ salmon wasn’t quite what we thought
- Why this rhino-zebra friendship makes perfect senseWhy this rhino-zebra friendship makes perfect sense
- When did bioluminescence evolve? It’s older than we thought.When did bioluminescence evolve? It’s older than we thought.
- Soy, skim … spider. Are any of these technically milk?Soy, skim … spider. Are any of these technically milk?
Environment
- Are the Great Lakes the key to solving America’s emissions conundrum?Are the Great Lakes the key to solving America’s emissions conundrum?
- The world’s historic sites face climate change. Can Petra lead the way?The world’s historic sites face climate change. Can Petra lead the way?
- This pristine piece of the Amazon shows nature’s resilienceThis pristine piece of the Amazon shows nature’s resilience
- Listen to 30 years of climate change transformed into haunting musicListen to 30 years of climate change transformed into haunting music
History & Culture
- Meet the original members of the tortured poets departmentMeet the original members of the tortured poets department
- Séances at the White House? Why these first ladies turned to the occultSéances at the White House? Why these first ladies turned to the occult
- Gambling is everywhere now. When is that a problem?Gambling is everywhere now. When is that a problem?
- Beauty is pain—at least it was in 17th-century SpainBeauty is pain—at least it was in 17th-century Spain
Science
- Here's how astronomers found one of the rarest phenomenons in spaceHere's how astronomers found one of the rarest phenomenons in space
- Not an extrovert or introvert? There’s a word for that.Not an extrovert or introvert? There’s a word for that.
- NASA has a plan to clean up space junk—but is going green enough?NASA has a plan to clean up space junk—but is going green enough?
- Soy, skim … spider. Are any of these technically milk?Soy, skim … spider. Are any of these technically milk?
Travel
- Dina Macki on Omani cuisine and Zanzibari flavoursDina Macki on Omani cuisine and Zanzibari flavours
- How to see Mexico's Baja California beyond the beachesHow to see Mexico's Baja California beyond the beaches
- Could Mexico's Chepe Express be the ultimate slow rail adventure?Could Mexico's Chepe Express be the ultimate slow rail adventure?