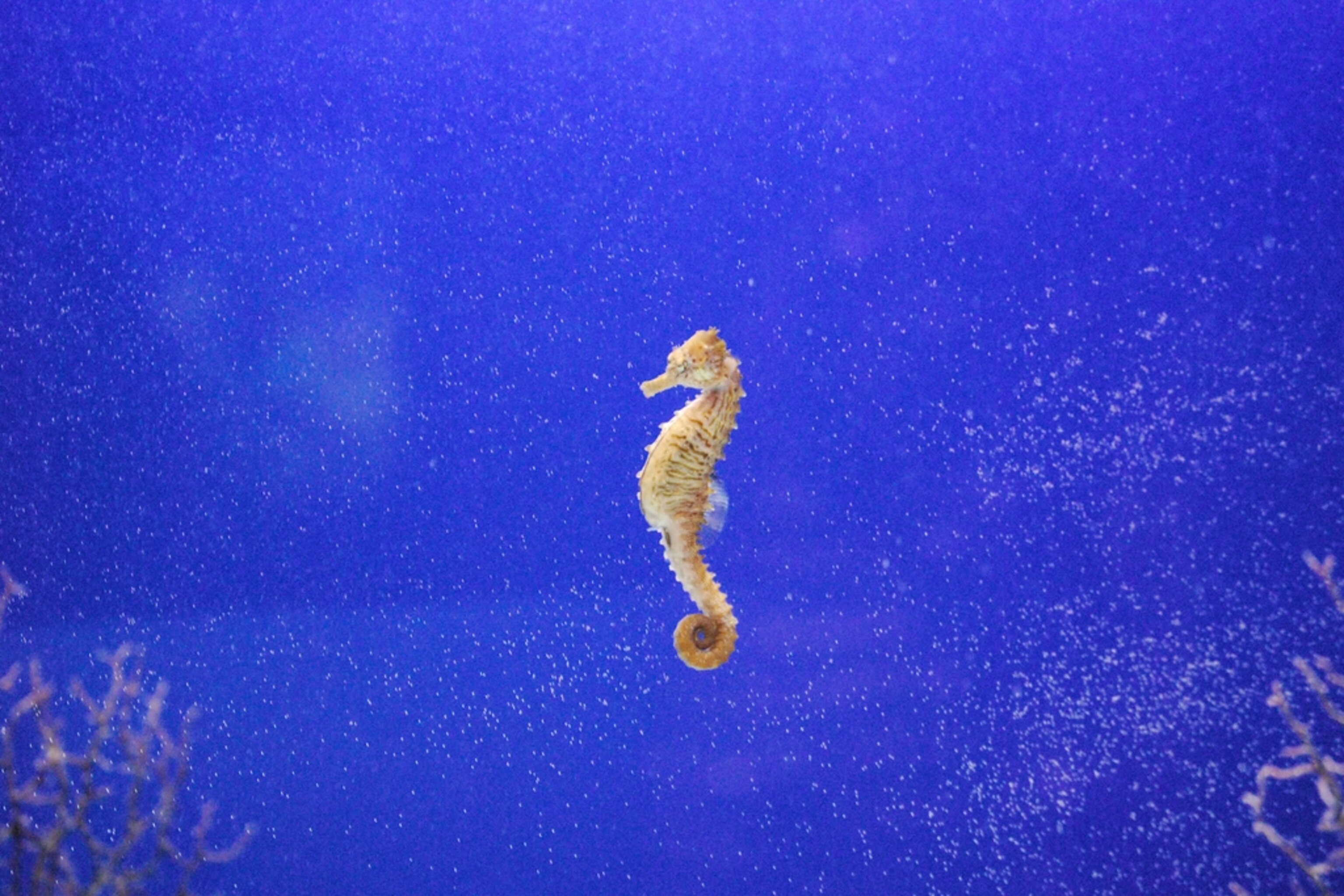
The Chatty Hippocampus
The hippocampus, a skinny log of brain tissue tucked in deep above your ear, is the star of memory research. People with damaged hippocampi — such as the famous patient Henry Molaison, known as H.M. — can’t make new memories. And studies in rodents have shown that creating new memories drives robust connections between neurons in the hippocampus.
Despite getting all the attention, the hippocampus doesn’t act alone. Our memories are as good as they are only because of the way the hippocampus talks to the rest of the brain. A study published this week in the Proceedings of the National Academy of Sciences underscores the importance of hippocampal interactions with the prefrontal cortex, the outer layers of brain located behind your forehead.
“As a field we focus so much interest on the role of the hippocampus, and rightfully so. But it means we haven’t investigated thoroughly the role of the prefrontal cortex and other areas,” says Adam Bero, a postdoctoral fellow in Li-Huei Tsai’s lab at the Massachusetts Institute of Technology, who led the study.
Bero’s study suggests a dynamic, back-and-forth relationship between the hippocampus and prefrontal cortex (PFC) during all stages of memory formation and storage. During the initial encoding of a memory, the PFC seems to drive activity in the hippocampus. Over the following days and weeks, when that memory is consolidated and stored, the information flow goes the other way, with the hippocampus driving activity in the PFC.
There’s been a lot of research linking the PFC to long-term memory storage. Rodent studies have shown, for example, that in the weeks after a new memory is created, the PFC increases and reorganizes its synapses, or junctions between neurons, adapting itself to permanently store that memory. But the PFC’s role in the initial creation of a memory has been less clear.
Brain-imaging studies have shown that the PFC lights up while people are forming new memories. And a couple of animal studies have dug into the issue on a molecular level. “But I think it would be fair to say that this [new] study is by far the most thorough,” says Richard Morris, a neuroscientist at the University of Edinburgh who led one of the older animal studies.
Bero’s team trained mice using a traditional ‘fear conditioning’ method, which works like this: The researchers move the animals from their home cages to a much larger chamber with a metal grid on the floor. After a few minutes of exploring this new environment, the animals receive two mild foot shocks and are then returned to their regular cages. “If we return the mouse to that [shock] chamber an hour, day, month or six months later, it will immediately exhibit freezing behavior,” Bero says. In other words, the unpleasant exposure causes the mouse to form a lasting fear memory.
The researchers found that an hour after forming that memory, the animals’ PFC shows dramatic changes in gene expression. The memory encoding dialed up the expression of 121 genes, many of which are related to changes at synapses that promote plasticity and learning. The experience also turned down the expression of 221 genes related to inflammation and the immune system, which are known to dampen synaptic plasticity.
The researchers then took a close look at individual neurons in the PFC an hour after fear learning. The cells changed their shape and function in ways that promoted electrical transmission. “These changes are typically thought to happen only weeks to months later,” Bero says. “Here we’re showing an early and rapid remodeling of the cortex after memory encoding.”
The most impressive part of the study used optogenetics, a popular new technique that allows researchers to switch brain cells on and off in an awake animal with a beam of light. Bero’s team showed that inhibiting PFC neurons during fear learning impaired their ability to form a long-term memory — when placed in the shock chamber 30 days later, they froze for less time than did animals whose PFC was not inhibited, suggesting that their fear memories were weaker.
“This is an exciting study,” says Paul Frankland, a neuroscientist at the Hospital for Sick Children in Toronto, who was not involved in the work. “Memory consolidation is a hugely dynamic process. Our memories for events and places may initially depend upon the hippocampus, but, with time, become increasingly dependent on the cortex,” he says.
It’s not totally clear, however, whether these changes in the cortex are caused by the creation of any episodic memory, per se, or rather are specific consequences of the fearful experience. “I suspect it is more likely that the mouse rapidly becomes scared of the context — because it gets shocked there — but doesn’t in any real sense remember an episode,” Morris says. The foot shock “is a pretty dramatic event in a mouse’s life, I assume,” Morris adds. “The prefrontal cortex may indeed wake up in such a scenario. But would it wake up every time? Or is this something that happens the first time that an animal is exposed to such a situation?”
There is no argument, though, that teasing out the interactions between the PFC and hippocampus will be critically important to our fundamental understanding of memory.
Intriguingly, the study may also have implications for understanding Alzheimer’s disease, Bero says. The PFC is one of the first brain areas to form the disease’s characteristic amyloid-beta plaques. If the plaques disrupt connections between the PFC and the hippocampus, he says, “it could be an early contributor to memory impairment in Alzheimer’s.”
Go Further
Animals
- How can we protect grizzlies from their biggest threat—trains?How can we protect grizzlies from their biggest threat—trains?
- This ‘saber-toothed’ salmon wasn’t quite what we thoughtThis ‘saber-toothed’ salmon wasn’t quite what we thought
- Why this rhino-zebra friendship makes perfect senseWhy this rhino-zebra friendship makes perfect sense
- When did bioluminescence evolve? It’s older than we thought.When did bioluminescence evolve? It’s older than we thought.
- Soy, skim … spider. Are any of these technically milk?Soy, skim … spider. Are any of these technically milk?
Environment
- Are the Great Lakes the key to solving America’s emissions conundrum?Are the Great Lakes the key to solving America’s emissions conundrum?
- The world’s historic sites face climate change. Can Petra lead the way?The world’s historic sites face climate change. Can Petra lead the way?
- This pristine piece of the Amazon shows nature’s resilienceThis pristine piece of the Amazon shows nature’s resilience
- Listen to 30 years of climate change transformed into haunting musicListen to 30 years of climate change transformed into haunting music
History & Culture
- Meet the original members of the tortured poets departmentMeet the original members of the tortured poets department
- Séances at the White House? Why these first ladies turned to the occultSéances at the White House? Why these first ladies turned to the occult
- Gambling is everywhere now. When is that a problem?Gambling is everywhere now. When is that a problem?
- Beauty is pain—at least it was in 17th-century SpainBeauty is pain—at least it was in 17th-century Spain
Science
- Here's how astronomers found one of the rarest phenomenons in spaceHere's how astronomers found one of the rarest phenomenons in space
- Not an extrovert or introvert? There’s a word for that.Not an extrovert or introvert? There’s a word for that.
- NASA has a plan to clean up space junk—but is going green enough?NASA has a plan to clean up space junk—but is going green enough?
- Soy, skim … spider. Are any of these technically milk?Soy, skim … spider. Are any of these technically milk?
Travel
- Dina Macki on Omani cuisine and Zanzibari flavoursDina Macki on Omani cuisine and Zanzibari flavours
- How to see Mexico's Baja California beyond the beachesHow to see Mexico's Baja California beyond the beaches
- Could Mexico's Chepe Express be the ultimate slow rail adventure?Could Mexico's Chepe Express be the ultimate slow rail adventure?