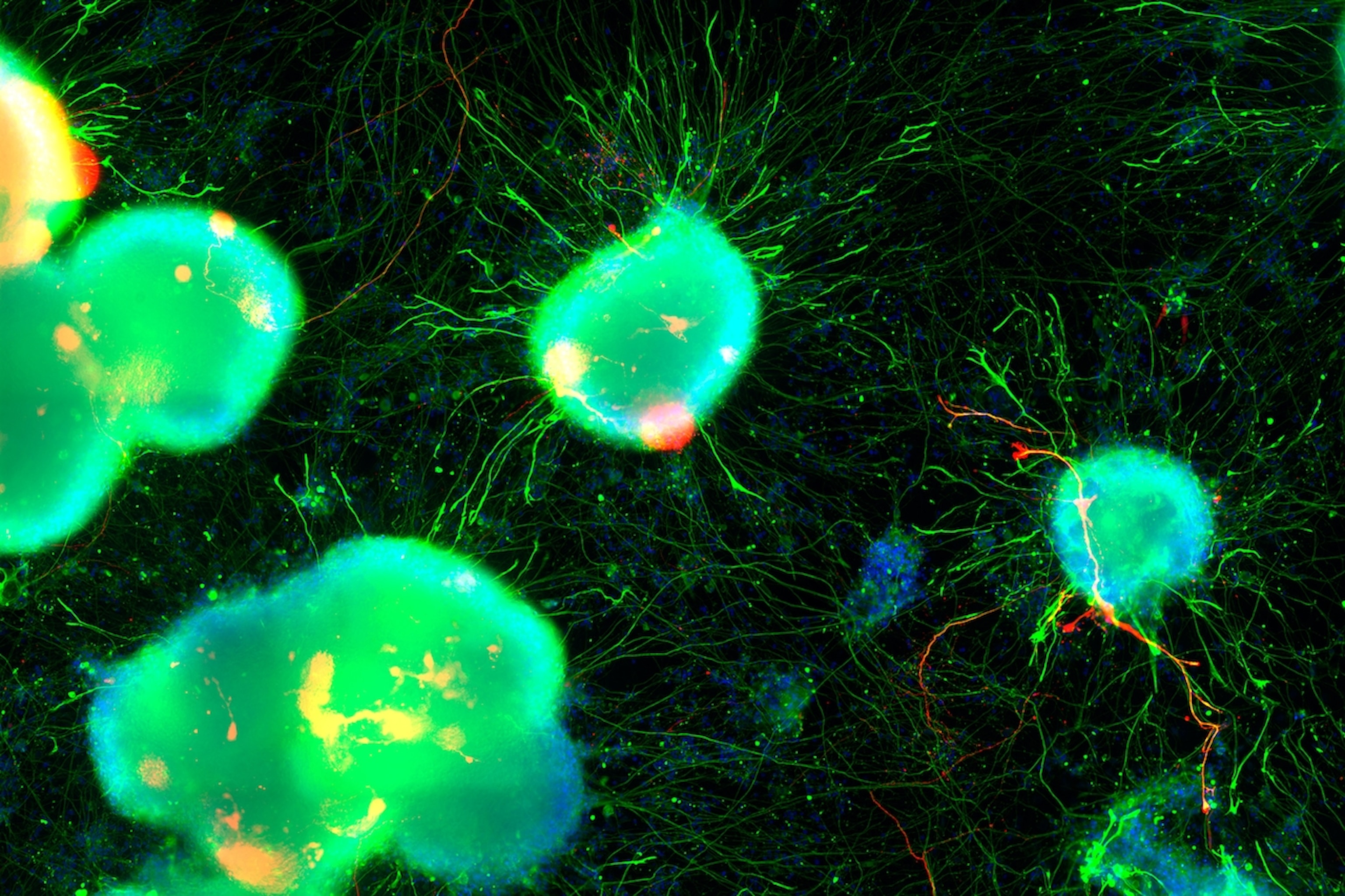
Lab Culture: The Tireless Brain Bot of the Finkbeiner Lab
This is the second edition of Lab Culture, a photo- and video-heavy series on the culture and methods of different labs. The first Lab Culture post was about the espresso machine and glowing fish brains in Florian Engert’s lab.
✼
The Brain Bot was born out of Steve Finkbeiner’s frustration. In the 1990s, as a postdoctoral fellow trying to understand why brain cells die in Huntington’s Disease, Finkbeiner spent hour after hour, day after day, in front of a microscope counting, describing, and cataloging cells in a dish.
At the time, researchers had known for a decade that Huntington’s — just like Alzheimer’s, Parkinson’s, and other so-called neurodegenerative diseases — is marked by characteristic protein clumps in the brain. But nobody could agree on what these clumps (known as plaques, tangles, and inclusions) were doing. Were they agents of destruction, triggering the cells (and, ultimately, the person) to die? Or were they incidental, accumulating in response to the disease but having no impact on its progression? Or were they actually a good thing, something the cell made to cope with getting sick?
To figure this out, Finkbeiner and his colleagues looked at rat neurons carrying the mutant protein that causes Huntington’s Disease. For each individual cell, the researchers would look at the shape of its nucleus and the length of its branches to determine whether it was healthy or sick. They did this over and over, for thousands of cells, and eventually the painstaking work paid off. In 1998, they reported in Cellreported in Cell that although the mutant protein does induce the death of neurons, this has nothing to do with the clumps of mutant protein that build up inside the cell. To the contrary, these inclusions slow down cell death, suggesting that the cell forms them as part of a protective strategy.
The study was controversial because it went against the predominant theory that inclusions cause disease, Finkbeiner says. “Some scientists in the field were skeptical and pointed out the limitations in the conventional techniques that were used, which made it impossible to be absolutely sure about the conclusions.”
Finkbeiner agreed that the methods had serious drawbacks. “With most cell biology, you study a handful of cells, take a few pictures, and draw your conclusions.” But those chosen cells may not be representative of the disease — in fact, sick cells die and disappear quickly, often eluding capture by researchers and their microscopes. “All you see is what remains. And it may be that what remains are the cells coping pretty well,” he says. What’s more, with typical methods researchers would watch cells for relatively short periods of time, on the order of seconds or minutes, even though neurodegenerative diseases take months or years to develop.
Traditional imaging methods also lacked objectivity, in Finkbeiner’s experience. While carrying out the study, he and his labmate often looked at the same cell and disagreed about how to characterize it. “If two collaborators sitting next to each other couldn’t agree, it made me discouraged about how to compare and reproduce results generated by different independent laboratories,” Finkbeiner says. “I wanted a system that would allow us to do 100,000 or a million cells, and to analyze those cells in an automated way, so you could take the human out of the loop.”
Enter the Brain Bot: a robotic microscope that can track changes in millions of cells over many months, if not longer. Finkbeiner began building it in 2000, after launching his own lab at the Gladstone Institutes in San Francisco, and has upgraded it several times since. The Bot has got to be the most assiduous member of the lab — it runs about 20 hours a day, seven days a week, and still, somehow, finds time for the occasional Tweet. The video below shows it in action. Using a robotic arm, the Bot automatically places a ‘well plate’ (a plastic tray with dozens of wells, each full of tens of thousands of neurons) under its microscope lens and takes pictures of the cells.
Because cells tend to stay still in the well, the microscope can keep track of each one over time. “The program basically gives each cell its own social security number” based on its position in three-dimensional space, Finkbeiner says. (Check out this review if you’d like to know more of the technical aspects of how it works.)
After building the Brain Bot, Finkbeiner went back to the old question about the protein build-up in cells in Huntington’s Disease. He carried out essentially the same experiment as before, putting the mutant protein in neurons and watching the effects over time. But this time, using the Bot, he could watch the same cell — actually, many cells — progress from sickness to death, and analyze whether this progression related to the accumulation of mutant protein in their nuclei. In some cells the process took 10 days, and the Bot captured it all. This work, published in Naturepublished in Nature in 2004, confirmed Finkbeiner’s previous experiment, showing that the protein clumps were part of a cell’s protective mechanism.
Finkbeiner has used the Brain Bot to study many other aspects of neurodegeneration (see slideshow above). The videos below show the neuronal branches of healthy mouse neurons (left) compared with those of cells carrying mutations in TDP-43, a gene linked to Lou Gehrig’s disease (right). Watching these branches, or neurites, grow is “very dramatic,” Finkbeiner says. “It is like growing a bowl of spaghetti. Those little cells can grow very, very long neurites.”
[embed width=”268″]http://vimeo.com/83342902[/embed] [embed width=”268″]http://vimeo.com/83342904[/embed]
More recently, Finkbeiner has been using the Brain Bot to track neurons made from induced pluripotent stem (iPS) cells. With iPS technology, researchers start with a small piece of skin from a living person. They then expose those skin cells to a certain chemical concoction that reprograms them back into a primordial state. Researchers then put those stem cells in yet another chemical soup that coaxes them to differentiate into a particular cell type, such as neurons. Some researchers, for example, have created at iPS neurons from people with Parkinson’s Disease who carry a mutation in the LRRK2 gene, which has been linked to the disease.
The trouble with using iPS cells to make neurons is that researchers end up with a mixed bag of cell types: neurons from all different brain regions, expressing different kinds of chemical messengers and in different stages of development. “We can’t make pure populations of any kind of neuron,” says Kristen Brennand, a neuroscientist at the Mount Sinai School of Medicine in New York who studies iPS neurons from people with schizophrenia. “That kind of heterogeneity has really limited the high-powered imaging because half of the cells you’re looking at are a different type than what you were hoping for.”
That isn’t much of a problem for Brain Bot, though, because it keeps track of individual cells. In a study out today in the Journal of Neuroscience, Finkbeiner and his postdoc, Gaia Skibinski, used the Bot to follow dopamine-producing neurons derived from people carrying the Parkinson’s LRRK2 mutation. Using this method, the researchers found something similar to what they had shown in the Huntington’s research. In Parkinson’s, mutant clumps of LRRK2 build up in the cell, but this accumulation doesn’t seem to be driving cell death.
Other researchers are impressed by the technique. “The single-cell analysis that they’re describing is not trivial,” says Brennand, who was not involved in the work. “It’s really powerful.”
Traditional methods track death rates by comparing the number of surviving cells of one type (say, Parkinson’s disease) versus another (normal cells), Brennand says. But this isn’t a great measurement because the dying process is slow. It takes awhile, in other words, for the cell counts of diseased cells to be significantly lower than the count of normal cells. Finkbeiner’s Bot, in contrast, can calculate an individual cell’s cumulative risk of death at any given time. “It’s a much more sensitive assay for death,” she says.
The Brain Bot’s automated, precise, and sensitive measurements are ideal for pharmaceutical research, as they can efficiently track the effects of an experimental drug, or many drugs, over time. And it’s not just for neurons. Finkbeiner has one project on brain cells called microglia, and is collaborating with a drug company interested in cancer stem cells.
The technology is progressing, too. Last year Finkbeiner teamed up with engineers from Google to make machine-learning algorithms that will help the Bot sift through ever-growing troves of imaging data. “Their vision of the future is pretty remarkable — that the human brain is a bottleneck,” Finkbeiner says, chuckling. “Maybe someday in the future I’ll just be a coffee cup plugging in the computer.”
*
Credit for the pretty cell images in the top slideshow goes to Dr. Julia Kaye/Gladstone Institutes.
Go Further
Animals
- How can we protect grizzlies from their biggest threat—trains?How can we protect grizzlies from their biggest threat—trains?
- This ‘saber-toothed’ salmon wasn’t quite what we thoughtThis ‘saber-toothed’ salmon wasn’t quite what we thought
- Why this rhino-zebra friendship makes perfect senseWhy this rhino-zebra friendship makes perfect sense
- When did bioluminescence evolve? It’s older than we thought.When did bioluminescence evolve? It’s older than we thought.
- Soy, skim … spider. Are any of these technically milk?Soy, skim … spider. Are any of these technically milk?
Environment
- Are the Great Lakes the key to solving America’s emissions conundrum?Are the Great Lakes the key to solving America’s emissions conundrum?
- The world’s historic sites face climate change. Can Petra lead the way?The world’s historic sites face climate change. Can Petra lead the way?
- This pristine piece of the Amazon shows nature’s resilienceThis pristine piece of the Amazon shows nature’s resilience
- Listen to 30 years of climate change transformed into haunting musicListen to 30 years of climate change transformed into haunting music
History & Culture
- Meet the original members of the tortured poets departmentMeet the original members of the tortured poets department
- Séances at the White House? Why these first ladies turned to the occultSéances at the White House? Why these first ladies turned to the occult
- Gambling is everywhere now. When is that a problem?Gambling is everywhere now. When is that a problem?
- Beauty is pain—at least it was in 17th-century SpainBeauty is pain—at least it was in 17th-century Spain
Science
- Here's how astronomers found one of the rarest phenomenons in spaceHere's how astronomers found one of the rarest phenomenons in space
- Not an extrovert or introvert? There’s a word for that.Not an extrovert or introvert? There’s a word for that.
- NASA has a plan to clean up space junk—but is going green enough?NASA has a plan to clean up space junk—but is going green enough?
- Soy, skim … spider. Are any of these technically milk?Soy, skim … spider. Are any of these technically milk?
Travel
- Could Mexico's Chepe Express be the ultimate slow rail adventure?Could Mexico's Chepe Express be the ultimate slow rail adventure?
- What it's like to hike the Camino del Mayab in MexicoWhat it's like to hike the Camino del Mayab in Mexico